CSULB Physicists Blow Up Molecules and the Mathematicians Pick Up the Pieces
We generally understand molecules and how they interact with each other – it's how we know whether an elastic band will stretch or snap, or whether a seatbelt will hold in a crash. But with new materials being developed out of molecules with more complex interactions, there's still a lot left to learn. That's where Professor Alex Klotz in Physics and Professor Ryan Blair in Math come in. They've been cooking something up – well, percolating something up, to be exact.
In an effort to study the physics of very large molecules to get a better understanding of how materials with similar molecules might behave, the CSULB Klotz Lab has been studying kinetoplast DNA from trypanosome parasites that cause tropical diseases like leishmaniasis. The kinetoplast is an unusual macromolecule that forms a network of thousands of circular molecules linked together like chainmail.
The stiffness of a molecule can change depending on its chemistry and its surroundings, and students in the Klotz lab, including recent master's graduate Josh Ragotskie, were interested in how the stiffness of kinetoplast changes when DNA loops are removed. To do this, they turned to percolation, using intense light to break some of the molecules, leaving the rest intact.
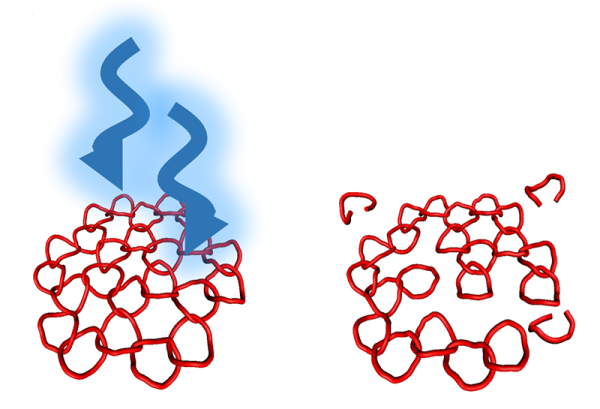
Making Connections
You've probably heard the term percolation with reference to making coffee, but the idea goes well beyond your local café. Percolation is the goldilocks condition of a system when its parts are connected neither too much nor too little. For kinetoplasts, percolation occurs when there are just enough connected molecules: any less and there aren't enough connections to hold it together.
The percolation threshold of kinetoplast DNA was unknown, so to figure it out Professor Klotz teamed up with Professor Blair to develop simulations that could determine this. Professor Blair, and student Nathaniel Morrison, start the simulations by removing tiles from different types of grids, keeping track of which tiles are still connected to the main grid. When the size of the main grid suddenly shrinks significantly, percolation has occurred.


So, did it work?
When it comes to research, the question that ends up being answered isn't always the one that is initially asked. In this case, both the experiments and the simulations ended up focusing on the extra loops that surround the edge of a kinetoplast, kind of like the elastic band in a hairnet.
We don't know what these extra loops do biologically, but as the network gets disintegrated, they end up holding it together longer than it would otherwise. In fact, the simulations showed that when these edge loops are included, the thicker edge of the network is the last thing to withstand percolation.
Explosive Reactions
But this needs to be supported with further experiments, so naturally these researchers turned to blowing things up…on a microscopic scale. Those experiments found that when the kinetoplasts explode, DNA fibers from the loops around the edge remain.

It may not have answered the initial question of how percolation affects the stiffness of a kinetoplast, but Klotz and Blair's investigation into edge loops was the subject of a recent paper in the Journal of Polymer Science.
"Overall, very creative and interesting work! I find the work interesting and helpful for the community interested in DNA topology," says Davide Michieletto, a researcher at the University of Edinburgh who studies the physics of kinetoplasts.
Let's go back to our original motivation: understanding complex molecules and the materials made from them. With our new knowledge of how these DNA structures disintegrate and leave behind a DNA polycatenane, a complicated chain of linked circular molecules, new experiments studying their properties in detail can now be undertaken.
Polycatenane molecules are complex, hard to make and hard to study, but they also may be an important part of future biomedicine, electronics and more. With the knowledge we have now, we can begin to understand complex molecules like never before.
Publication
The effect of the kinetoplast edge loop on network percolation. J Ragotksie, N Morrisson, C Stackhouse, RC Blair, AR Klotz. Journal of Polymer Science (2023).
About the Klotz Lab
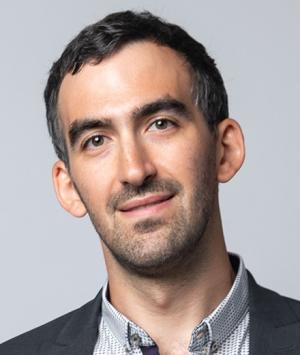
Dr. Alex Klotz is an assistant professor in the Physics and Astronomy Department at CSU Long Beach. The research group studies phenomena at the interface of biological physics and soft condensed matter.
Dr. Klotz is interested in using tools provided from biology to answer questions about the physics of soft materials.
Learn more: Klotz Lab
About the Blair Lab
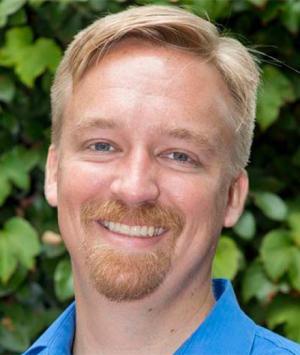
Dr. Ryan Blair is an associate professor in the Mathematics and Statistics Department at CSU Long Beach. The research group studies low-dimensional geometry and topology with an emphasis on knot theory.
The world in which we live is three dimensional. This basic fact implies that we live in some 3-manifold. In this way, the study of 3-manifolds illuminates the nature of the universe. Knotting phenomena in manifolds is so rich that a complete understanding of it would lead to a complete understanding of all 3-manifolds. The broad goal of Dr. Blair's research is to study 3-manifolds via knots.
Dr. Blair's recent research investigates the structure of 3-manifolds and the knots they contain by applying modern techniques such as thin position and distance in the curve complex to classical constructions such as Heegaard splittings, Dehn surgery, and diagrammatic knot invariants.
Learn more: Blair Lab